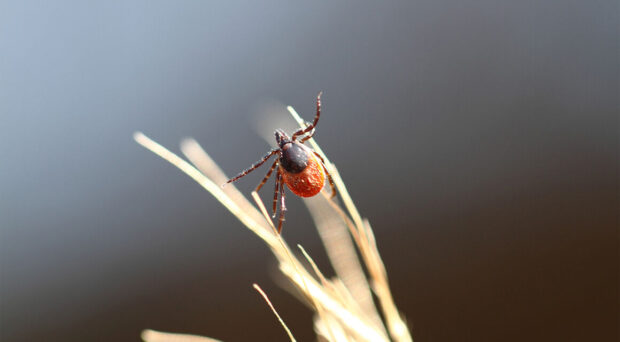
Picture walking through a blooming meadow, flowerheads bobbing against your legs, skin lightly brushing the long grass; an idyllic thought. Another creature enjoys this concept: the tick. From their patient perch on vegetation, they will latch onto passersby that did not have the forethought to wear longer clothes. But without a direct hit, they will be left stranded. Their stumpy, little legs are incapable of jumping, so finding a new passenger requires mechanical contact. Even a small gap would have been an insurmountable chasm.
However, a team of researchers remembered that, in everyday life, tiny objects can ‘leap’ millimetres of distance and adhere to charged surfaces. Static electricity surrounds us, whether it is through loose hair sticking on our skin, a nasty sting opening your car, or a real threat to ignitable fuel. Why would this principle not apply to ticks, minute creatures smaller than a peppercorn? Sam England, Katie Lihou, and Daniel Robert sought to test the role this could play in permitting ticks to find new hosts.
They chose the castor bean tick (Ixodes ricinus) as their test subject, an insect found across a swathe of Eurasia including much of the EU. Using both acrylic sheets and rabbit feet, charged by rubbing against one another, they demonstrated that the ticks would be readily attracted to the targets across distances as far as 2cm (see video).
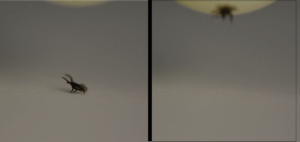
The nymph stage of the insect, the slightly immature form used in these experiments, is around 11mm long when unfed. So this small leap is equivalent to traversing 18 body lengths unassisted. In human terms, this would be akin to spontaneously propelling across 30m of empty space, nearly to the top of a ten-story building.
Following on from these slightly cruder methods, the team used an electrode to finely control the electrostatic potential between tick and target, this time only a few millimetres away from one another. By varying the voltage and altering the distance between the subjects, the experimenters were able to calculate the voltage needed to attract a starving nymph-stage tick across any theoretical distance. Based on their estimations, a 750V potential (described as a conservative estimate of vertebrate skin charge – based on studies of humans, geckos, snakes, and hummingbirds) should be able to attract a tick across 3mm of space. The extreme estimates of human surface charge (30 kV) would be enough to zip a tick several centimetres.
The tick itself does not induce its own charge for this process, as both positive and negative static charges are equally able to pluck the bugs from their substrate. This suggests that the electrostatic attractor itself induces the tick’s opposite surface potential (by repulsion of the same charges in nearby objects). Interestingly, the researchers noted that the ticks could disrupt these tests by holding on tight to the base plate and refusing to move. Future experiments could investigate whether ticks ‘choose’ when to let the static charges of the environment take them for a ride, based perhaps on sensing their proximity to a new host.
The electric field of an animal is not uniform, meaning that there will be areas more likely to attract ticks than others. To investigate this, the researchers, rather amusingly, created an electrostatic model of a cow in a field to find where the surface potentials are at their strongest. Dr England stated that “The geometry of a cow is very complicated”, which begets interesting electrical dynamics.
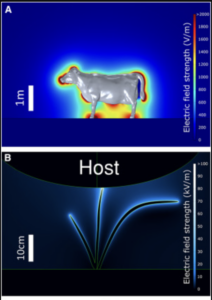
The ruminate topology suggests that the extremities will be most at risk (the snout, legs, and tail), and indeed these body parts are the ones that will come closest to vegetation and the vying invaders. Assuming that the grass is grounded, with zero net charge, this will create the requisite electric fields needed for electrostatic tick carriage.
It remains to be seen whether electromagnetic transportation applies to other tick species, however there is no theoretical reason why it would not. After all, static electricity is a universal phenomenon. Indeed, there is no need to limit this conclusion just to ticks. The investigators point out that lice, fleas, and mites are easily small enough to be carried this way. These electric charges may indeed be used by the tick to detect their hosts passing nearby, instigating questing behaviour (in which the ticks reach out from plant surfaces and grope for their victim). Electroreception by arthropods has already been found in bees and spiders. The castor bean tick itself is of central importance to public health as it is a vector for a frightening roster of afflictions: lyme disease, tick-borne encephalitis, babesiosis, tularaemia, among others. This includes bacterial, viral, and parasitological conditions. By using this species as a model, the researchers have already shown the relevance of their work to vector-borne disease control.
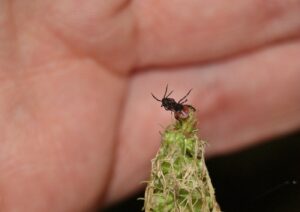
So what can we do about it? Friction against vegetation may make it harder to minimise static, but the team at Bristol University point out that anti-static coatings or fabrics can be used to reduce the chances of ticks being pulled in by our clothing. Coatings could also be applied directly to the fur of livestock or pets to help protect them. Static electricity may be everywhere, including across our own skin, but it isn’t unavoidable. Perhaps this will shut a door to tick transmission, even if it is a small one.
Comments