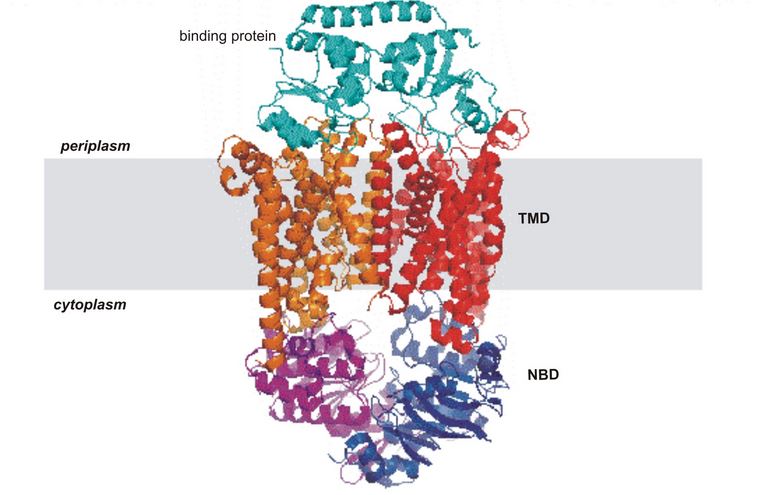
Great expectations: structural biology, macromolecular machines and moving targets
It is no news that the technical developments of recent years have made possible enormous advances in the understanding of complex or dynamic macromolecular systems. But as David Stuart remarked in the course of the inaugural Astbury Conversation, recently held in Leeds, great advances have led to correspondingly great expectations. Here is a selective account of how these expectations have been met, as reflected in talks from the Symposium.
Viruses, vaccines and drug targets
David Stuart was speaking on his work on picornaviruses – causes of much human and animal disease, perhaps most infamously polio and foot and mouth disease – and the goal of delivering vaccines and antivirals based on an understanding of the structural features and requirements of viral pathogens – a long-term aim of his lab.
Confronting the same challenge, Peter Stockley demonstrated the impact of cryo-EM on the issue of viral packaging. Virus capsids assemble around viral RNA specifically, and using cryo-EM with a battery of other techniques he has been able to identify the signals that provide this specificity and show how they do this, with a view to novel drug targets.
Better together
Peter Stockley’s work was just one example of a major theme of the meeting – how combining datasets from the panoply of biophysical methods – NMR, crystallography, mass spectrometry, molecular dynamics and both light and electron microscopy – which do not quite tell the full story on their own can enable data that are incomplete and may be conflicting to become informative.
The ordering of subunits in the TriC chaperonin had been an open question, for instance, with relatively low-resolution mass spectrometry data and crystal structures giving different answers, until the work described by Plenery speaker Michael Levitt.
The ordering of subunits in the TriC chaperonin had been an open question, for instance, with relatively low-resolution mass spectrometry data and crystal structures giving different answers, until the work described by Plenery speaker Michael Levitt.
He used these data to inform molecular dynamics simulations which showed that the ordering of the subunits differ from what these lower resolution data had indicated, and provide a better resolution of the structure.
The structural asymmetry he and his colleagues found through their work also helped unravel the mechanism of the protein, suggesting that similar subunits could play different roles: with some being ‘slightly cracked’ to bind substrates for folding and some functioning mostly as ATPases.
In a more obviously translational application, Erin Cutts spoke on how a combination of microscopy and molecular dynamics methods made it possible to propose a molecular mechanism for one of the major hazards of infection with the malaria parasite.
The knob-associated protein KAHRP is required for the formation of ‘knobs’ on red blood cells in malaria. These ‘knobs’ result in endothelial adhesions that prevent infected red blood cells from being swept into the spleen for clearance along with their passengers, and involve interactions of KAHRP with other parasite proteins and the red blood cell protein spectrin.
By applying both computational and microscopy Erin Cutts and colleagues have been able to show that the positive charge on the surface of the K2 domain of the protein attach it to spectrin and the cytoskeleton, and how this leads to spiral structures at the centre of these adhesion-causing protrusions.
Moving targets
One of the properties of the proteins in the knob complex that makes them such a challenge is their large content of structurally disordered regions, a focus of much current interest and which have traditionally been difficult to analyze.
These and protein species with similarly elusive properties, such as folding intermediates, have become approachable both with combinations of techniques that include molecular dynamics simulations and with technical developments in NMR spectroscopy.
The Astbury Centre’s director Sheena Radford described some of her investigations on the mechanisms of amyloid formation and its cellular toxicity.
The Astbury Centre’s director Sheena Radford, for example, described some of her investigations on the mechanisms of amyloid formation and its cellular toxicity. She showed how new NMR techniques for capturing transient folding intermediates of b2 microglobulin can explain why some variant forms of the protein are amyloidogenic and that hexameric aggregates can form and disrupt cell membranes.
NMR spectroscopy has historically been limited by protein size, with large complexes not providing informative spectra, but Lewis Kay showed a way round this in how he demonstrated allostery in the regulation of the massive, and hence ‘nearly invisible’ to NMR, proteasome complex.
By specific labelling of a subset of the amino acids in the complex, NMR spectra could be generated to show how the chemical environment of these residues in one subunit of the complex changed depending on the presence of other subunits, indicating a degree of allosteric regulation and helping to understand the mechanisms of this vital cellular machinery.
NMR is more readily used to target proteins at the other end of the size spectrum, and their dynamics over time, and Anais Cassaignau used these strengths effectively in a novel strategy to study the folding process of a nascent Ig domain directly from the ribosome with small, isotopically labelled fragments.
She showed that chemical signatures of folding of the Ig domain of FLN5 appear after significant amounts of the protein emerge from the ribosome, suggesting that folding is suppressed during translation to avoid mis-folding, and also identified some interactions between nascent chains and the surface of the ribosome which may be partly responsible for this.
‘The lazy option’
Michael Levitt claimed in his plenary talk that he chose physics as the ‘lazy option’, requiring only an understanding of principles and applying mathematics rather than memorising of pathways, chemicals and facts.
Biophysics could be seen as the worst of both worlds, requiring as it does both an involved understanding of thermodynamic and kinetic principles and an in-depth knowledge of the interactions of proteins and their domains, and then their relationship to physiology. But the rewards are enormous.
Thomas Wylie
Latest posts by Thomas Wylie (see all)
- What is a Pseudoenzyme, and why? - 26th September 2016
- Great expectations: structural biology, macromolecular machines and moving targets - 27th May 2016
Comments